Research Overview
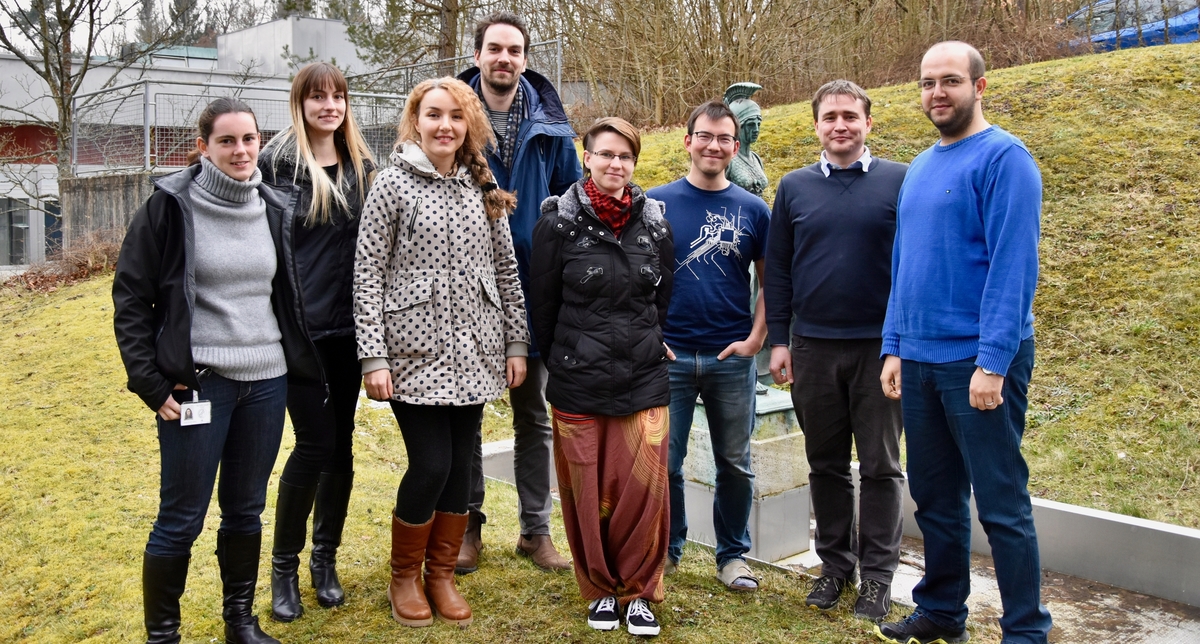
Animals outperform legged robots albeit limited muscle power density, low sensorimotor conduction velocity, and slow reflexes. Yet we observe that animals run faster and more efficiently, and incorporate higher agility in a smaller building volume. So how does the animal's neurocontrol orchestrate limbs and body, and what role plays morphology? How are animals not overwhelmed by a control task that otherwise requires high-end computers, kilo-hertz rate sensory feedback, and complex mathematical models in robots? We develop robots and models to explore these questions and understand the underlying principles of dynamic locomotion in animals and robots.
We are intrigued by the biomechanics of legged locomotion; animals run agile yet energy-efficient, high-performing yet robust. The study of animal and human movement has traditionally been approached through biomechanical gait analysis and, more recently, through multi-body simulations.
While the collection of biomechanical data remains the gold standard in the field—utilizing force plates, motion tracking, and muscle activation recordings—fundamental aspects of locomotion remain challenging to study with these conventional methods. Examples are the underlying neural control patterns, their closed-loop structures, the complex dynamics of muscles and tendons, and the interactions of large networks of compliant, closed-loop tendon structures.
At the Dynamic Locomotion Group, we develop robotic models to replicate hypothesized mechanisms and control principles of legged locomotion. By implementing these models in robots and conducting experimental validations, we aim to provide evidence for the fundamental principles governing legged locomotion. An example:
Through cadaver studies, we observed that the leg joints of large birds exhibit mechanical coupling, with particularly intriguing toe motion in ratites. We hypothesized that toe orientation during stance and swing plays a critical role in engaging and locking the leg’s intrinsic compliant mechanism.
To test our hypothesis, we developed a bird-inspired robotic leg featuring a compliant toe-locking mechanism, informed by mathematical models and mechanical adaptations. The resulting BirdBot robot, powered by only four actuators, exhibits natural and dynamic leg motions like to those of large birds. Despite receiving minimal, sparse locomotor control inputs, the robot achieves locomotion with a record-low cost of transport [].