Muscles, control, and damping in legged locomotion
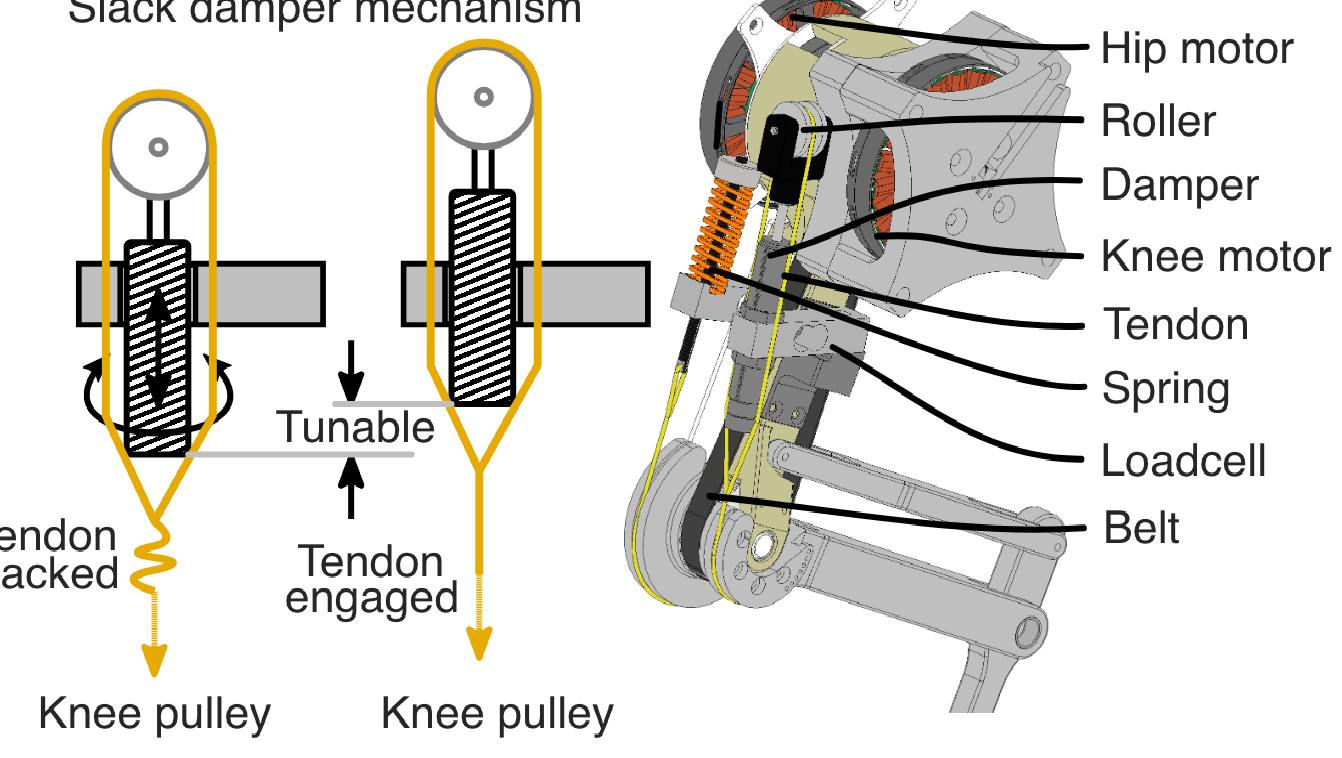
Neuromuscular control loops operate with substantial communication delays, yet animals exhibit remarkably robust locomotion even under adverse conditions. This robustness is puzzling, as axonal conduction velocities are limited to a few tens of meters per second, whereas robotic systems transmit signals effectively at speeds approaching that of light. If reflex loops deliver sensory feedback with significant delays, one might expect sensorimotor control to become destabilized.
It has been hypothesized that both neural control and muscle mechanics contribute to locomotion robustness. This explanation assumes a hierarchical structure in which low-level adaptive mechanics and high-level sensorimotor control work together to mitigate the effects of transmission delays.
To investigate this, we developed a tunable physical damper system [], consisting of a tendon with adjustable slackness connected to a physical damper. This slack damper mechanism allows control over damping force, activation timing, effective stroke, and energy dissipation. We tested this mechanism on a legged robot operating in open-loop mode. The auto-engaging slack damper activates during perturbations, enabling perturbation-triggered damping, which enhances robustness with minimal energetic cost. This finding provides a new functional interpretation of redundant muscle tendons in animals as tunable damping systems.
Further insights come from in vivo muscle experiments and musculoskeletal simulations, which suggest that the muscle preflex—an immediate mechanical response to perturbations—plays a crucial role. Preflexes act within a few milliseconds, an order of magnitude faster than neural reflexes. However, their short duration makes them difficult to quantify in vivo.
In collaboration with the research groups of Daniel Häufle and Tobias Siebert, we aim to quantify the mechanical work performed by muscles during the preflex phase (preflex work) and investigate their role in force modulation []. Additionally, we examined the influence of fiber viscosity arising from the force–velocity relationship. Using a novel approach, we isolated and quantified the preflex force produced by the force–velocity relation in musculoskeletal simulations [
]. Our analysis focused on muscle responses to ground-level perturbations during simulated vertical hopping, specifically within the first 30 milliseconds after impact, a critical period where neural feedback is too slow to contribute.
Video