Enhanced Flexible Mold Lifetime for Roll‐to‐Roll Scaled‐Up Manufacturing of Adhesive Complex Microstructures
Bioinspired Microstructured Adhesives with Facile and Fast Switchability for Part Manipulation in Dry and Wet Conditions
Smart Materials for manipulation and actuation of small-scale structures
3D nanofabrication of various materials for advanced multifunctional microrobots
Liquid Crystal Mesophase of Supercooled Liquid Gallium And Eutectic Gallium–Indium
Machine Learning-Based Pull-off and Shear Optimal Adhesive Microstructures
Information entropy to detect order in self-organizing systems
Individual and collective manipulation of multifunctional bimodal droplets in three dimensions
Microrobot collectives with reconfigurable morphologies and functions
Self-organization in heterogeneous and non-reciprocal regime
Biomimetic Emulsion Systems
Giant Unilamellar Vesicles for Designing Cell-like Microrobots
Bioinspired self-assembled colloidal collectives drifting in three dimensions underwater
Mobile Microrobotics
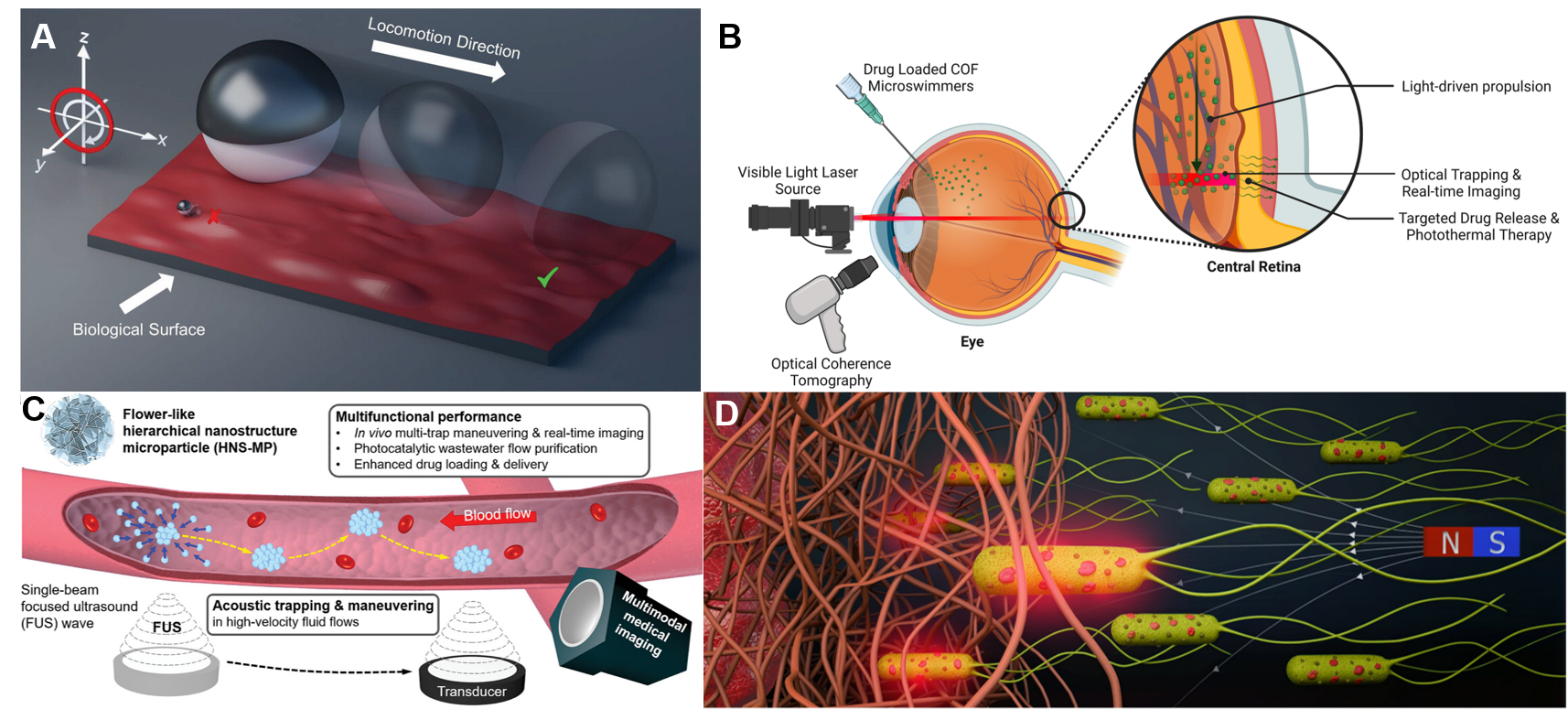
Our research addresses the critical challenges of developing microscale mobile robots, focusing on two primary approaches that push the boundaries of miniature robotic systems.
The first approach involves biohybrid microrobots, which ingeniously integrate genetically engineered microorganisms, such as bacteria and algae, with robotic structures. This innovative design enables remarkable capabilities such as self-propulsion and targeted cargo transport. We have demonstrated significant success in magnetic steering bacteria-powered red blood cells and synthetic drug-loaded particles through sophisticated sensing mechanisms like chemotaxis, aerotaxis, and pH-taxis. The most compelling achievement is an on-demand drug delivery system that increases effectiveness by four orders of magnitude compared to the passive release of the drug molecules from carrier particles.
Our second approach centers on synthetic mobile microrobots, which can be actuated through three primary methods: magnetic, optical, and acoustic. Magnetic actuation leverages external magnetic fields, offering long-range capabilities and high tissue penetration, though it requires bulky auxiliary equipment. The approach includes subtypes such as torque-driven, force-driven, and shape-deformable microrobots. Optical actuation provides exceptional precision by utilizing optical forces, making it particularly suitable for biotechnology applications, but it is limited to transparent environments, such as intraocular space. Acoustic actuation emerges as a versatile method, employing ultrasound waves to enable non-invasive, deep tissue penetration in non-transparent media using single or multiple focused ultrasound probes to trap the ultrasound-responsive particles.
Looking forward, our research aims to combine these actuation methods, improve microrobot autonomy, and enhance imaging systems for precise feedback control. The ultimate goal is to expand potential applications across medicine, biotechnology, and micromanufacturing, creating increasingly sophisticated microscale robotic systems that can interact dynamically with their environments.